[Author’s Note: This was a hard topic to tackle. Research in the field of epigenetics is brand new and most studies are done at the cellular level, rather than as applied research. My hope in writing this article is to provoke thought that there is much to exercise than what we see on the surface. Exercise is possibly the most powerful drug that we have at our disposal and it has the potential to greatly impact our lives. Keep running!]
Deoxyribonucleic acid, or DNA, is a double strand helix molecule that contains the genetic instructions that make up an individual. Each person has a unique genetic code that makes them who they are. DNA can be thought of like a blueprint for a living organism containing the instructions for building the organism. Segments of DNA grouped together, called genes, code for a particular protein within the organisms. Genes are expressed through the processes of transcription and translation, where the genes are first copied and then synthesized into a protein, respectively.
Our DNA determines much than just our physical appearance. It also determines how we function as an organism, including health and susceptibility to disease. We are each born with a unique genetic code, which will ultimately determine the expression of our inherited traits. We can’t change our genes… or can we?
The study of epigenetics examines how genetic modifications can change gene expression. These modifications resulting in gene expression are accomplished without altering the DNA sequence. How is that possible? Well, as the genetic code is copied (transcribed), epigenetic modifiers can bind to the DNA sequence to ‘change’ the code. This modification often occurs through methylation, a process in which methyl groups bind and ‘block’ any genes in that stretch of DNA. (See Figure 1.) The result is that genes in that sequence will are switched ‘off’ or not expressed. Thus, epigenetic modifiers have the ability to alter gene activity simply by blocking or not blocking the genetic code as it’s being copied.
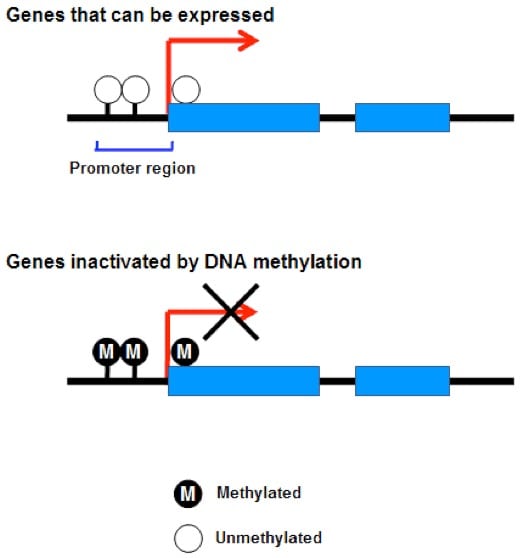
Figure 1. Inactivation of a gene by DNA methylation.
Source: National Cancer Center Research Institute, 2010.
So, what does that mean? In plain English it means that our genes are not set in stone. Not all genes are active at all times. As you can imagine, epigenetics is a growing area of interest because of the potential implications for health. Recent studies have shown that not only do epigenetics influence your genes, but also can be passed down from one generation to the next. For example, eating too much may cause obesity but can also predispose your kids to be obese, even before they are conceived.
And so, what does this have to do with exercise? Well, lifestyle factors such as nutrition and exercise have been associated with methylation of specific genes leading to various health outcomes. The expression of specific genes is not just determined by our DNA, but also by our lifestyle. This goes back to the age-old debate of nature versus nurture. Are we the way we are because of our genetics or because of lifestyle? The lifestyle we lead may affect us more than we know. For better or worse, our bodies adapt to the environment they are placed in.
Exercise and Health
The importance of exercise for health has been well established. We know that exercise lowers risk for chronic diseases and also helps maintain energy balance for weight management. However, this seems to be just the tip of the iceberg. Recent studies have also linked exercise to methylation of specific genes related to risk factors for chronic disease. For example, exercise has been associated with methylation of genes responsible for the secretion of pro-inflammatory cytokines, which are the leading cause of chronic inflammation. Methylation, or blocking, these pro-inflammatory genes reduces the risk for cancer (Zhang et al., 2011).
In terms of obesity, exercise can actually change the way fat is stored in the body. A recent study investigating a six-month exercise intervention on DNA methylation in previously sedentary men found altered response in adipose tissue. The methylation occurred in genes related to obesity and type 2 diabetes (TCF7L2 and KCNQ1), meaning these genes were silenced following the exercise intervention. The exercise intervention consisted of a weekly group-training program of one 60-minute spinning session and two 60-minute aerobics sessions per week. On average, the subjects attended 1.8 sessions/week (Ronn et al., 2013). The major finding from this study is that endurance exercise positively impacted fat storage and metabolism, lowering the risk for obesity and type 2 diabetes. These changes were present in those with and without family history of type 2 diabetes and obesity. In this case, nurture seems to trump nature.
The relationship between exercise and chronic disease is difficult to measure is human populations, but a link has been clearly established. Beside the recent epigenetic studies, empirical evidence observing lifestyle factors in disease-free populations have found regular exercise to be a common theme. In a study assessing the link between physical activity and risk of breast cancer, a cohort of 25,624 women were followed for 13.7 years. In this study, those with greater leisure-time physical activity were associated with a lowered risk of breast cancer (Thune et al., 1997). Although we are far from fully understanding the dynamic influence of exercise, the importance of exercise for optimal health and avoidance of disease is becoming even more apparent.
You might be wondering how much exercise you need to gain these positive benefits. It’s great to have such positive results from daily exercise, but if it entails running hours each day, then it’s not very practical for the average person. The reality is that very little exercise is needed to cause these changes. A recent study investigating different levels of physical activity, 26 to 30 minutes/day versus less than 10 minutes/day, found that those who accrued more had more DNA methylation. Although this finding was not statistically significant, it suggests that more physical activity produces results in greater methylation and very little physical activity is needed to cause these changes (Zhang et al., 2011). Despite the lack of strong evidence, this study still shows the potential for exercise, in as little as 30 minutes/day, to positively influence epigenetic modifiers.
There are two major take-home messages from these studies: 1) endurance exercise not only improves health at the cellular level, but also is associated with methylation of genes, further lowering risk for chronic disease, and 2) the amount of exercise required for these changes is not astronomical. This may have huge implications for public-health recommendations. Not only is exercise important, but every little bit counts. Surely most people have time to set aside 30 minutes per day to exercise, especially knowing the potential health benefits established in studies like these. Many more studies are needed to fully understand the implication of exercise on epigenetics, however the association between exercise and positive epigenetic modifications is clear.
Exercise and Performance
Can these epigenetic changes as a result of exercise also impact performance? It appears that exercise can, indeed, promote changes that will benefit performance and not just lower risk factors for disease. A recent study looking at skeletal muscle found changes in DNA methylation before and after an acute bout of exercise. Post-exercise, fewer methyl groups were found on some of the genes involved in energy metabolism (PGC-1a, PPAR-õ, PDK4). Conversely, genes unrelated to energy metabolism remained methylated, or blocked (Barres et al., 2012). This means that, in response to a single exercise session, genes related to the breakdown of fuels for energy were expressed while the genes not involved in energy metabolism were blocked. Energy metabolism is an important part of performance and the more efficient a body is at converting foodstuff to energy, the better for performance. Demethylation of genes related to energy metabolism produces a favorable adaptation in the skeletal muscle, including increased fat and glucose metabolism, improving the potential work capacity of the skeletal muscle. An even more interesting finding from this study was that the changes in the skeletal muscle were intensity dependent, meaning the greater the intensity the greater the gene demethylation (Barres et al., 2012). Taken together, this clearly illustrates the stress-adaptation process of the body. The more stress, or exercise, placed on the body, the greater the adaptation.
Are these epigenetic changes permanent? If someone spends a couple months engaging in exercise training, will the positive epigenetic changes sustain long after the activity is ceased? Unfortunately, it doesn’t appear so. Epigenetic changes are a response to an environmental stress. Since DNA is not altered, when the environmental stress is removed, the DNA code will revert back to its natural state. Just as the body becomes de-trained when exercise is discontinued, epigenetic modifiers revert back to their original state without the influence of exercise. This does shed light on the age-old dilemma of ‘nature versus nurture,’ however. Although DNA determines the original programming, lifestyle appears to have a big influence on the genes expressed that make us who we are. We may be born with a set of genes, but what we do in our day-to-day lives can determine what genes are expressed.
Is There A Sports Gene?
There have been some recent reports that elite athletes may have a special gene, a sports gene, which allows them to reach a high level of fitness. This idea seems to explain why some people can dedicate their whole lives to training and still only be mediocre while others seem to have a natural, innate ability. What makes elite athletes different? Is it their work ethic and dedication to the sport or are they born with something that predisposes them to be great? David Epstein explores this possibility in his book titled The Sports Gene. In his book, Epstein examines both genetic factors and lifestyle influences that may contribute to an athlete’s success.
In one instance, Epstein examines a Finnish cross-country skier named Eero Mäntyranta who has won seven Olympic medals in cross-country skiing (between 1960 and 1972). Mäntyranta has a genetic defect, primary familial and congenital polycythemia (PFCP), that causes increased red blood cell mass and hemoglobin. This mutation to the erythropoietin receptor (red blood cell) can increase the oxygen-carrying capacity of the blood by up to 50%. In endurance sports, this is a huge advantage as one of the limiting factors is oxygen delivery to the working muscles. Mäntyranta’s genetic mutation produced the same effects in performance as blood doping. Mäntyranta’s success in skiing is easily attributed to his unique genetics, but that’s not the whole story. The other part of the story, Mäntyranta’s upbringing, also explains much of his success. As a child, Mäntyranta grew up in a poor household and was forced to ski an hour each way to school every day. It was in doing this trek that Mäntyranta realized he could be a successful skier and avoid a life of poverty. So which was more important for Mäntyranta’s success: nature or nurture? In his book, Epstein suggests that it’s both nature and nurture that make an athlete successful. You can’t separate one from another; they are both equally important is molding individuals into the kind of people they are (Epstein, 2013).
A review of current genetic factors in elite athletes has suggested that up to 66% of the variation of the athletes genetics are non-modifiable. Meaning, that over half of the genes that make elite athletes different are what they are born with, rather than as a result of their lifestyle (Eynon et al., 2011). This review also points out that genetic variants are complex, including gene-gene interactions, rare genes, or polymorphisms, which may also influence athletic performance. Just because we begin to understand one aspect of genetic influences doesn’t mean we understand the full picture. And with so much individuality it’s difficult to pinpoint a clear understanding of how genetic influences impact an athlete’s success.
As my college ski coach used to say, “There are many Olympians sitting on bar stools.” Indeed, there are probably many individuals who will never know their athletic potential because they’ve never trained hard. So, what really matters more? A drive to work harder than anyone else or a natural-born ability? I don’t think we really have an answer. It seems that someone who possesses both qualities would have the highest potential. In racing, however, it doesn’t always end with the best ‘on paper’ athlete crossing the finish line first. In fact, that’s why we race. People can do extraordinary things, despite adversity. This is also part of what drives our passion and excitement for sports, seeing someone come from behind or defy the odds to accomplish the impossible is inspiring. It gives us hope that maybe we can also be great. Maybe not having the natural-born ability inspires more passion and drive to work hard. Who would win a race between an athlete with natural-born ability or one with a fire inside? I don’t know… and that’s precisely why it’s exciting to watch races unfold. It always amazes me how sports are not just physical. Passion, drive, and perseverance can really impact performance.
Summary
Although we can’t change our DNA, we do have the ability to control our lifestyle, leading to epigenetic changes that can influence us both positively and negatively. We already know that exercise is important for health, but now we are learning that exercise might benefit us even further. There are still a lot of unknowns in the field of epigenetics. Scientists are at the tip of the iceberg when it comes to understanding genetics and lifestyle. It does seem, however, that the more we learn, the more nurture or lifestyle seems to play in determining the characteristics of an individual. Lifestyle factors, such as diet and exercise, have a deeper role in health and fitness and may offer even further benefits at the cellular level. From a performance standpoint, exercise appears to induce epigenetic changes within skeletal muscle, improving energy metabolism. These changes are intensity dependent and occur in response to even a small bout of exercise. Further, it appears that elite athletes may be born with a set of genes that predisposes them to be great, but they become great because of their lifestyle. Passion, drive, and hard work are perhaps just as important to success as a natural-born ability. So the answer to the nature versus nurture debate is still out. It appears that both factors impact us and may be equally important in determining who we are.
References
- Barres R, Yan J, Egan B, Treebak JT, Rasmussen M, Fritz T, Caidahl, Krook A, O’Gorman DJ, Zierath JR. Acute exercise remodels promoter methylation in human skeletal muscle. Cell Metab 2012;15(3):405-11.
- Cloud J. Why genes aren’t destiny. TIME, Jan 6, 2010.
- Epstein D. The sports gene: inside the science of extraordinary athletic performance. Penguin Group, 2013.
- Eynon N, Ruiz JR, Oliveira J, Duarte JA, Birk R, Lucia A. Genes and elite athletes: a roadmap for future research. J Physiol 2011;589(13):3063-70.
- National Cancer Center Research Institute. DNA Methylation. [broken link removed]
- Ronn T, Volkov P, Davgardh C, Dayeh T, Hall E, Olsson AH, Nilsson E, Tornberg A, Nitert MD, Eriksson KF, Jones HA, Groop L, Ling C. A six months exercise intervention influences the genome-wide DNA methylation pattern in human adipose tissue. PLOS Genetics 2013;9(6):1-16.
- Sanchis-Gomar F, Garcia-Gimenez JL, Perez-Quilis C, Gomez-Cabrera MC, Pallardo FV, Lippi G. Physical exercise as an epigenetic modulator: eustress, the “positive stress” as an effector of gene expression. J Stren Cond Res 2012;26(12):3469-72
- Thune I, Brenn T, Lund E, Gaard M. Physical activity and the risk of breast cancer. New Eng J Med 1997;336(18): 1269-75.
- Zhang FF, Cardarelli R, Carroll J, Zhang S, Fulda KG, Gonzalez K, Vishwanatha JK, Morabia A, Santella RM. Physical activity and global genomic DNA methylation in a cancer-free population. Epigenetics 2011;6(3):293-9.